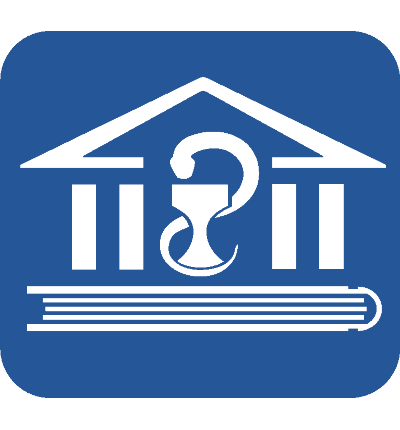
REVIEW
Ethical issues in pediatric clinical trials
1 Pavlov First Saint Petersburg State Medical University, Saint-Petersburg, Russia
2 St. Petersburg State University, St. Petersburg, Russia
Correspondence should be addressed: Aleksey S. Kolbin
ul. L’va Tolstogo, 6–8, Saint-Petersburg, 197022, Russia; аur.liam@niblok.xel
Author contribution: Emelianova LI — literature review, writing an article; Kolbin AS — article editing.
RELEVANCE
Historically, many drugs, widely applied in pediatric practice, have not been properly studied in children. The drugs often lack complete data on safety, effectiveness and dosage in children, and, thus, the consequences of their prescribing are not known fully. As a rule, pediatricians are well aware of the lack of information about the use of the drug in children in many information leaflets. In this case, ethical, legal, economical and other considerations can result in refusal from potentially important drug-induced pediatric treatment with these drugs.
Another option available to a healthcare professional consists in using both unlicensed (not registered in pediatrics) and off-label (other than as intended) drugs [1]. As it was mentioned above, ethical aspects belong to one of the reasons of limited study of drugs. It should be noted that ethical issues arise almost at any stage of studying drugs in children, from clinical trials (CT) including pilot studies (study of microdosing, study of one dose at subtherapeutic doses or within the assumed therapeutic range, study of multiple doses), to extrapolation.
History of clinical trials in children
In the beginning of 1960, the world has faced significant changes in regulation of drugs. The thalidomide tragedy was the reason [2, 3]. This resulted in Kefauver-Harris amendments to the U. S. Food and Drug Act in 1962, and formation of national systems of spontaneous reports about adverse events. The positive effect of introducing the reporting system consists in additional control of drug safety. However, the changes designed to ensure a safer drug-induced pediatric therapy had an opposite effect as well [4]. Thus, according to Kefauver-Harris amendments, to obtain an approval for entering the pharmaceutical market, a drug should be both safe, and have significant advantages over other drugs. The evidence should be presented to the Food and Drug Administration (FDA) for review.
The unintended consequence of the act taken in good faith is described the best by Shirkey H, a founder of pediatric pharmacology, in 1968. He used a term ‘therapeutic orphans’ to describe the existing situation when the majority of drugs presented in the market are not labelled as ‘for use in children’ though the drugs are actually widely used among children as first-line therapy [5].
Until 1997, the world has seen a few studies on development of drugs in pediatrics. Thus, data on proper dosing, safety and effectiveness, that could be used while prescribing the majority of drugs to children, were lacking for decades. Serious consequences of prescribing off-label drugs in children and slow acceptance of children by the society as participants of clinical trials paved the way for legislative initiatives in the U.S. and Europe [6]. Thus, the Food and Drug Administration Modernization Act was adopted in the U.S. indicating that a manufacturer or holder of a marketing authorization can get additional 6 months of exclusive sale of its product if there is an official request from the FDA to conduct pediatric studies [7].
From the clinical and scientific point of view, advantages of this act include a systemic mechanism for pediatric studies of novel drugs, establishment of effectiveness, safety and pharmacokinetic basis for use and dosing among children (from premature children to adolescents), and an incentive to search for the best ways for such trials.
Moreover, to overcome the shortage of drugs for pediatric use, coordinated efforts have been taken during the last 20 years, including development of national and international research networks for pediatric trials, and changes in the process of drug approval by regulating authorities. The taken measures promoted not only expansion of knowledge about the approved drugs but also urged manufacturing companies to include children into clinical trials of drugs that can be used in pediatrics in the future.
The Best Pharmaceuticals for Children Act, BPCA, adopted in 2002, and the Pediatric Research Equity Act, PREA, subsequently adopted in 2003, can serve as examples. Both BPCA, and PREA were updated in 2007 in accordance with the FDA Amendments Act, and became an essential part of the FDA Safety and Innovation Act in 2012. The Regulation on Medicines for Pediatric Use is a European equivalent of the act. The regulating authorities on both sides of the Atlantic have a right to request from the companies that submit applications for new drugs to present a detailed plan of the trial (Pediatric Study Plan in the U.S., Pediatric Investigation Plan in Europe) for the drugs that can be used in children [8]. Subsequently, over 1,200 pediatric trials were submitted to the FDA, with the majority of them being submitted since 2007. The 21st Century Cures Act, adopted in the U.S. in December 2016, accelerates development of novel medical products and contains some provisions that expand the ability to upgrade the plans of clinical trials and assessment of clinical outcomes, making the process of drug approval easier [7].
The Last Reauthorization FDA Act of 2017 expands the programs of the Best Pharmaceuticals for Children Act related to the trials of unpatented pediatric drugs until 2022 [9]. On the one hand, these changes create problems for drug manufacturers. This occurs because historically, inclusion of children into clinical trials was not a part of planning of drug development. It was difficult to perform so due to a number of reasons including ethical issues and issues associated with acceptability, rare occurrence, standardization, endpoints, safety, dosing and feasibility.
On the other hand, innovative developments such as new design of clinical trials, in silico pharmacology (pharmacometrics modelling) and microdosing method have been introduced into the process of drug development in recent decades. Adoption of these acts significantly accelerated intensive growth in the area of development of neonatal and pediatric drugs. Since 1997, a number of conducted clinical trials in children has increased by more than 5-fold; about 29,000 clinical trials in pediatrics and neonatology have been conducted by the end of 2022 [10, 11]. From February 1998 to May 2023, FDA approved 1049 amendments in pediatric drug labeling. It means addition of novel information about safety, effectiveness or dosage for novel and already applied drugs [12]. At the same time, no pediatric dosing recommendations are found in 2021 with the 9-year lag in pediatric instructions after approval of instructions for adults [13].
Microdosing in pediatrics — pilot pediatric trials
The guideline developed by the International Council for Harmonization of Technical Requirements for Pharmaceuticals for Human Use, ICH, M3, states that microdosing is a first-in-human trial, where drug exposure is less than that in phase I trial (less than the maximum tolerated dosage); it doesn’t aim at producing a therapeutic effect and is not intended to assess tolerability [14]. The term ‘an exploratory clinical trial’ has been suggested. The European Medicines Agency, EMA, and FDA determine a microdose as 1/100 of the minimum predicted therapeutic dose obtained with extrapolation from the preclinical phase of animal trials or as a dosage not exceeding 100 mcg of the studied drug (or 30 nmol for biological ones) depending on what dose is the least. No therapeutic, toxic or radiotoxic doses (radioisotope labeling) are expected during exposure of such low doses. Though microdoses do not produce a serious effect and cause no effects in the body, various pharmacological effects produced by them can be detected using targeted approaches and sensitive analytical methods. Some authors refer to this type of studies, apart from exploratory clinical trials, as to phase 0 [15]. Basic barriers to using microdosing studies (exploratory studies or phase 0 approaches) among vulnerable population groups, and among children in particular, are of ethical nature and associated with safety. Three basic safety issues include drug exposure, procedural burden and radiation exposure. In microdosing studies, drug exposure is considered subtherapeutic and is identified as a minimal risk only. It represents a significant advantage over studies with therapeutic doses in vulnerable groups of population. In the pediatric population, the procedural burden is mainly associated with a number of blood samples. The WHO Guideline recommends to limit the volume of blood sampling, taking at least 3% of the total blood volume during 1 month and at least 1% of the total blood volume within 24 hours. The issue of procedural burden in a 3 kg newborn can be taken as an example. 1% of the total blood volume will be equal to 2.4 ml (1/100 of 240 ml). This blood volume can be taken from the patient within 24 hours. It can easily meet demands for sampling with liquid chromatography/mass spectrometry (LC/ MS): 100 mcL per sample. Accelerator mass-spectrometry with a higher sensitivity also decreases the sampling requirements: 2 mcL per sample in total, depending on drug concentration.
Radiation exposure is low in PET, and extremely low in AMS, corresponding to the normal background exposure [15]. M. Turner et al. (2015) calculated radiation exposures using microdosing methods in children. The radioactive dose was calculated using the worst half-life scenario of 40 days, with radioactive exposure ranging from 0.33 to 0.8 µSv.
It is much lower than the annual background exposure (2.5 µSv in the Netherlands), air travel within the European area (1–15 µSv), computed tomography (CT) of the head (1200 µSv) or chest X-ray (12 µSv). Thus, radiation exposure, which can be obtained during studies with AMS, does not exceed the minimum risk [16].
The study of microdosing in children is reviewed in the document entitled ‘Additional warranties for children in clinical trials’ cited in the FDA rules [17]. The principle of scientific necessity and risk assessment is described in this document. The ethical principle of ‘scientific necessity’ arising from the FDA rules states that pediatric studies are a must only if data obtained during the study refer to an essential need of public healthcare in pediatrics and can’t be obtained from adults. The ethical principle requires to decrease the risks for subjects by way of excluding unnecessary procedures. The basic goal of pediatric clinical studies regulated by the FDA is to establish the dosage, safety, effectiveness of the studied drugs to an extent sufficient for licensing of both children, and adults. Additional means of protection of children included into the study consist of two basic categories: 1) in the lack of any perspective of getting a direct benefit for the included child, the studied product or procedure should represent at least an insignificant increase as compared with a minimal risk (i. e., a lower risk) in accordance with 21 CFR 50.51 / 21 CFR, or 50.53), or 2) the studied product or procedure should represent a perspective of direct benefit enough to substantiate higher risks (i. e., ‘a higher’ risk path in accordance with 21 CFR 50.52). In the last case, direct benefit should be obtained by a study participant and arises from a certain study intervention or procedure. As during the microdosing studies the administered dose of the studied drug is not sufficient to provide a therapeutic effect, the studies do not present an opportunity to obtain a direct benefit for a child. Thus, the microdosing studies should be assessed following the low risk. According to regulatory acts of the U.S., there are two categories of studies with a lower risk such as a minimal risk or insignificant increase as compared with minimum risk [4]. The minimum risk is defined as a ‘probability and value of harm or discomfort expected in a study, which do not exceed those commonly found in a daily life or while accomplishing regular physical or mental examinations or tests’ [4]. (Insignificant increase as compared with minimal risk can be allowed if additional criteria are followed). Intervention or procedure approved for this category should ‘provide generalized knowledge about a subject’s disorder or condition, which is essential for comprehension or improvement of the subject’s disorder or condition’. Assessment of whether the intervention or procedure is just an insignificant increase as compared with the minimum risk should be done using enough data (for instance, any study-related pain, discomfort or stress won’t be serious). The ‘disorder or condition’ is determined by the Institute of Medicine (IOM) as a set of ‘specific physical, mental, psychomotor or social features’, which, as per our scientific data or clinical knowledge, threaten a child’s health or ‘increase the risk of health problems in the future’ (IOM). So, a child can be healthy, but subject to the risk of a disorder or condition taken as a study object.
The ethics of administration of subtherapeutic doses to children during studies of novel drugs has been discussed by the Pediatric Ethics Subcommittee (PES) of the Pediatric Consultation Committee created on May 11, 2011. Some factors that influence the risk assessment, including the quality of available data obtained during animal studies or how well the drug was characterized in adults, a child’s age as related to the age of the population where the drug was studied, and the necessity of data obtained in adult studies of dose-dependent and dose-independent toxicity have been reviewed [18]. It has been decided that studies of subtherapeutic dosing can be conducted within the pediatric population if the preliminary favorable data were obtained after animal studies and studies of dose-dependent and dose-independent toxicity. So, pediatric microdosing studies should be of a scientific and social significance to correspond to the approval criteria. Second, as a microdose is insignificant to produce a therapeutic effect, pediatric microdosing studies should not be viewed in accordance with 21 CFR 50,52 (when direct benefit can be obtained by a study object). However, a drug microdose corresponds to the criteria of insignificant exceeding of a minimum risk and can be studied in children with a disorder or condition (disease or its risk), indicated by a study object. The FDA approves the use of microdosing studies in pediatrics. However, the issue of whether the microdosing studies can be approved among healthy children is still unsolved [19]. Nowadays, several microdosing pediatric studies have been conducted in the U.S. and Europe. The doses varied from 3 to 30 ng/kg with a set being 20 mcL per sample. The levels of administered radioactivity were extremely low. A linearity between the microdosing range and therapeutic dose was shown in the studies with such drugs as Ursodiol, Midazolam and Acetaminophen. The obtained data testify in favor of subsequent studies of other drugs and involvement of other vulnerable groups of population into studies. Of special interest is studying ontogenesis in children, especially metabolism, transport and excretion of drugs while changing the functional activity of organs using the microdosing method.
Thus, in the study of Mooij MG et al. (2017), a significant decrease of the relative rate of paracetamol sulfation has been demonstrated; intense glucuronidation processes during the first 6 years of life after single peroral administration of Acetaminophen have been confirmed. Thus, the effect of age on perorally administered metabolism of Acetaminophen has been studied in a minimal risk among children [20].
First pediatric dose
It is rather complicated to determine the first pediatric dose while developing drugs, because both effectiveness, and safety should be taken into account. It is unreasonable to administer a non-effective dose to a child (except for microdosing studies). Allometric scaling (when applicable), pharmacokinetics simulation, including physiologically substantiated pharmacokinetics models and pharmacokinetics/ pharmacodynamics simulation, are used in clinical studies to improve dosing recommendations [21]. Ideally, every drug used in pediatrics should have pharmacokinetic and pharmacodynamic profiles, that will be reflected in guidelines on dosing and information leaflets. Unfortunately, the majority of pediatric drugs are currently lacking an evidence pharmacokinetics/pharmacodynamics basis. This results in the lack of empirical data to select a dose in people under 18 and significant variability in the amount and quality of data containing dosing recommendations. Many dosing recommendations are based on adult and animal data extrapolation in combination with various scaling principles. In the view of the existing situation, the principles of allometric scaling are currently taken as the best of the affordable means to select the most exact dosing regimen among 2-year-old children. Thus, they have to be mentioned in dosing guidelines. According to the study conducted by Chitty KM et al. (2018), while analyzing the Australian Medicine’s Handbook Children’s Dosing Companion and British National Formulary for Children, it has been found out that dosing was done for 2% and 3.4% of drugs respectively using methods of allometric scaling [22]. The approach based on the dosage depending on body weight (mg/kg) is prevailing in the recommendations (about 2/3 of all analyzed drugs). In some cases, use of this approach results in more than two-fold deviation from the doses calculated with allometric scaling. This can be especially important for drugs with a narrow therapeutic range, where an exact dose assessment is necessary. Digoxin is an example of drugs with a very narrow therapeutic range. When the dosage of digoxin is calculated using the method of allometric scaling, the variability between the minimum and maximum doses occurs to a far lesser extent than the one observed when dosage is calculated based on the body weight. Thus, the risk of drug-induced toxicity can be minimized when a more exact dosage of Digoxin is selected [22].
Ethical issues of involving children in research
Participation of children in scientific studies has always been a subject of heated discussions and, as a consequence, a constantly changing field. As soon as the importance of research ethics and informed consent has been established, the debates about ethical issues associated with participation of children in studies continue in the U.S., Europe, Canada, etc. Historically developed opinion that has been predominant for a long time was that children should not participate in studies of drugs mainly for ethical reasons. In recent decades, a view on the problem has changed. The prevailing current opinion is that children deserve to participate in clinical studies of high quality and ethical standards, and obtain access to the drugs approved respectively. There is an argument stating that drug studies in children are essential to obtain evidence of safety and effectiveness of drug-induced therapy and cooperate in development of drugs against widely spread and essential pediatric diseases. Thus, ethical concepts that allow and even encourage participation of children in studies have been currently formed. It is increasingly being discussed that participation in studies won’t be more than a minimum risk for a child. Though there is less probability that children will participate in phase I studies (except for children with oncological diseases), they will definitely have an ethical right to participate in phase II and phase III studies.
Another ethical conflict in pediatrics states that a consenting person (a parent or guardian) is not a person who receives therapy. There are growing calls for obtaining consent not just of parents, but also of children, especially adolescents, for ethical approval. At the same time, it is still unclear how informed consent to participation in the study can be solved best due to contradictions related to what a consent is and at what age the consent can and must be obtained. In many jurisdictions, the minors can provide an informed consent to various medical interventions, including the ones associated with a significant risk. However, they often fail to provide consent to participation even in studies with a very low risk. The aspect is still an area of active debates and discussions [23].
The draft Informed Consent Information Sheet Guidance for IRBs, Clinical Investigators, and Sponsors and draft Ethical Considerations for Clinical Investigations of Medical Products Involving Children contain the following recommendations: while taking a decision whether children are capable to provide consent, it’s necessary to take into account the age, maturity and mental condition (mental abilities and stage of development) of children who plan to participate in the study. It is frequently believed that children aged 7 years and more can provide consent. The child does not have to gain a complete understanding of a clinical study to provide the consent if the child is able to understand interventions and associated procedures (for instance, blood sampling for analysis).
In accordance with these guidelines, children’s consent is not a necessary condition for a clinical trial if: 1) children’s capabilities are so limited that consultations can’t be provided, or 2) intervention or procedure associated with a clinical trial can result in an indirect benefit, which is essential for children’s health and well-being, and is available in the context of a clinical trial only. Under these circumstances, requirements to parental consent are preserved.
Meanwhile, even if it is established that children can provide consent, it may be unnecessary in the following cases: 1) a clinical trial is associated with a minimum risk for the subjects 2) refusal won’t produce a negative effect on the rights and well-being of the subjects; 3) it’s almost impossible to conduct a clinical trial without a refusal; 4) when appropriate, the subjects will be given additional respective information after their participation [24, 25].
The issue of acceptability
The issue of acceptability concerns a child’s family, doctors, medical organizations, research centers and researchers. Historically, it was believed that parents are not willing to register their children in clinical trials. Based on the results of the conducted trials, it was assumed that the situation was rather apparent than obvious. An English and Canadian trial, and a trial held in France revealed that pediatricians who have not undergone ethical training, were unwilling to include children into clinical trials [4]. It becomes more obvious that children are interested in participation in the trials due to altruistic reasons and for the benefit of other children. The trial comfort level in children can be significantly different in various institutions. Regional and national pediatric trial networks, which can be the sources of standards and resources to improve developments and conduct clinical trials in children, can be a possible solution in this case. The National Institutes of Health Pediatric Pharmacology Research Network uniting research subdivisions in the U.S. can serve as an example. The Medicines for Children Research Network in the United Kingdom created by the National Healthcare Service and combining the experience in the area of pediatric research in the Great Britain should be mentioned as well. The Network created in the Great Britain was united with the Pediatric Specialty Group to create a clinical practice society generating the national experience in pediatric trials. It makes possible to exchange experience and practice [23].
The Pediatric Cluster organized in August 2007 by EMA and FDA is an example of international cooperation. The cluster represents exchange of information about drug development for children in the form of daily teleconferences between the regulating authorities of various countries. The objective of teleconferences is to ensure that all pediatric trials are held in compliance with strict scientific and ethical standards and that all pediatric patients are not subject to unnecessary (duplicate) trials.
The Pharmaceuticals and Medical Devices Agency joined the conferences in November 2009, whereas the Ministry of Health of Canada did the same in September 2010. They acted as observers. Nowadays, they are active participants of these monthly data exchange. The Australia’s Therapeutic Goods Administration joined the teleconferences in January 2014 being an active participant till now.
Monthly discussions include such issues as ethics and safety of pediatric trials, discussion of protocols, plans of pediatric trials, selection of efficacy endpoints, status of the current pediatric trials, outcomes of pediatric trials, plans of long-term safety monitoring, etc. This cooperation provides for safe ethical and scientific basis for these trials in children.
Clinical trials in neonatology
Review of 1,081 registered trials in children has shown that 74% of these trials were held in children elder than 2 years old. At the same time, changes in drug distribution and reactions to the drugs commonly predominate in children under 2 [26]. To protect children from unexpected unfavorable consequences in the majority of pediatric programs, researchers and regulating authorities select sequential developments, starting from trials for elder children and ending with trials for younger children.
However, following more than decade-long experience, a protective effect of using the sequential development has not been proven yet. At the same time, evidence of harm due to a long-term use of off-label drugs within the most fragile population (younger children) is indisputable [27]. In newborns, having clinical trials is a complex issue due to several reasons. There are knowledge gaps in the area of clinical pharmacology of newborns.
A few patients in neonatal trials can make it difficult to interpret the results of pharmacokinetics, pharmacodynamics or dose-ranging studies. It is even more complicated because the first month of life sees the growth, rapidly changing physiology and maturation of drug-associated receptors, metabolizing enzymes and transporters. These factors enable significant inter- and intraindividual variability of pharmacokinetics and pharmacodynamics, often observed in newborns [28]. Trials in premature newborns is even a more complicated issue due to unique pathophysiology and reaction to therapy within the population. Though about 200,000 premature newborns are annually admitted to intensive care units, very few drugs for therapy of this group of patients have been studied and approved. Drug development trials in newborns can be costly, risky and have ethical or practical limitations. In 65% of cases, intensive care units use not licensed and off-label drugs. As a rule, only one out of 10 drugs most frequently used at intensive care units is intended for premature children.
It means that irregular assignments, insufficient dosing, overdosing and unique or more frequent or more severe adverse effects are common in this vulnerable group [26]. In accordance with the Guidance on Clinical Trials of Drugs in Pediatrics adopted by the International Conference on Harmonization of Technical Requirements on Registration of Pharmaceutical Products for Human Use, studying drugs in premature newborns requires proper protocol development with participation of experts in neonatology and pharmacologists [24]. In rare cases, effectiveness of trials in adults and even in elder children can be extrapolated to premature newborns. Premature newborns are not a homogeneous group of patients. Body mass and gestational age can vary significantly, producing an effect on pathophysiology and reaction to drug-induced therapy [29]. For instance, a newborn who was born at 24 weeks gestation and is not under 4 weeks of age has physiology, which — during the first days of life — is different from the one of a newborn who was born at 28-weeks [30].
Thus, important features that should be taken into account with these patients during clinical trials include as follows: gestational age at birth and age after birth (corrected age); immaturity of renal and hepatic clearance mechanisms; protein (especially bilirubin) binding and exclusion; penetration of drugs into the central nervous system (CNS) due to the brain-blood barrier immaturity; unique diseases of newborns (respiratory distress syndrome of a newborn, open arterial duct, primary pulmonary hypertension); unique susceptibility of premature newborns (for instance, necrotic enterocolitis, intraventricular hemorrhage, retinopathy of the newborns); rapidly changing maturation of all physiological and pharmacological processes resulting in various dosing regimen in long-term administration of drugs, and increased transdermal absorption of drugs and other chemical substances. The issues of trial design that should be taken into account include as follows: weight- and age-related stratification (gestational and postnatal ones); small volumes of blood (40 ml of blood in a newborn with 500 g of weight); a limited number of patients; and difficulties in outcome estimation [29].
Out of 1,043 changes in the labelling of drugs approved for use in pediatrics by the FDA from 1999 to 2022, only 79 were allowed to be used among newborns. It should be noted that changes in drug information can be introduced even if no trials on newborns were conducted. For instance, safety information based on non-clinical data (for instance, data obtained in animal trials) can be included. Thus, out of 79 drugs with information about their use among newborns, only 57 underwent trials involving the age group [31].
A special amendment stimulating to conduct respective trial in newborns was proposed to the FDA Safety and Innovation Act adopted in 2012. In 2015, Wang J et al. studied databases of the FDA and found 43 drugs studied in newborns from 1998 to 2014. Twenty of them were approved to be used in newborns [28]. For 10 drugs, the approval was based on effectiveness data in newborns supplemented by pharmacokinetic data for four drugs. Approval for newborns was based on complete extrapolation of data from elder patients for six drugs, whereas partial extrapolation served as a basis for four of them. The majority of drugs studied in newborns were intended to treat infectious diseases (44%). Proton pump inhibitors to treat gastroesophageal reflux disease were the second most common ones (28%). Four drugs (famotidine, remifentanil, rocuronium and fenoldopam) out of those approved for use in newborns were tested with the dosing range and endpoints of pharmacodynamics to select the dose for subsequent phase III trials of effectiveness. Only three drugs (meropenem, linezolid and lucinactant) had dosing recommendations for premature newborns. Linezolid and meropenem required various dosing intervals for premature and mature newborns due to differences in pharmacokinetics within these two groups, whereas lucinactant was approved in premature children only. The label for the three products clearly states that these drugs are contraindicated in premature newborns because of their toxicity (lopinavir/ritonavir), non-effectiveness (nitrogen oxide to prevent bronchopulmonary dysplasia) or a lack of trials to support dosing recommendations (sevoflurane) [28].
Failures in the pediatric clinical trials
J. Momper et al. (2015) show that 42% of pediatric trials conducted from 2007 to 2014 failed to establish neither safety nor effectiveness of the studied drugs [32]. Thus, 44 unique drugs presented for review to the FDA were not labeled as approved for pediatric use. The main reasons for failures during pediatric trials included insufficient effectiveness (38 drugs, 86%) and safety issues (7 drugs; 16%). Bioanalytical deficiency (saquinavir trial) and a lack of the delivery system necessary for young patients (fluticasone/salmeterol trials) were classified as other failures. It has been established that properly selected dose was a failure in ten trials where effectiveness was not established. Effectiveness was not displayed in 8 drug development programs due to impossibility to take into account basic differences in diseases of adults and children. Actual examples include differences in immune tolerance in children and adults (adefovir trial), differences in ADP platelet aggregation test (clopidogrel), differences in the role of acid in GERD pathogenesis (esomeprazole, lansoprazole, omeprazole and pantoprazole), differences in manifestations in case of herpes simplex virus (famciclovir) and differences in hypertension etiology (eplerenone). It was established that the rate of failures in pediatric trials is doubled up, when complete effectiveness extrapolation from adults is impossible. Knowing the natural anamnesis of a pediatric disease is essentially important to develop pediatric trials and select the basic effectiveness endpoint [32]. Improper or incomplete comprehension of pathophysiology of many conditions hampers detection of clinically significant biomarkers of pharmacodynamics. An example can include the use of gastric pH as an activity marker of proton pump inhibitors in treatment of symptomatic gastroesophageal reflux disease in newborns. It is currently comprehended that though newborns may have some signs and symptoms of gastroesophageal reflux disease, including regurgitation, vomiting and dyspnea, they probably have no gastroesophageal reflux disease mediated by acidic gastric content. Meanwhile, acidic gastric content is the basic pathophysiological factor of gastroesophageal reflux disease in elder children and adults. Symptoms of a gastroesophageal reflux disease in newborns are mainly associated with motility and abnormal temporary relaxation of the lower esophageal sphincter. The lower esophageal sphincter becomes mature by 34 weeks and occurs postnatally in newborns born before 34 weeks. Complete maturation of the sphincter occurs within 13 months. Besides, pH suppression in newborns can be irrelevant as the gastric pH in newborns can exceed 4 [28]. As a result, differences in pathophysiology of symptomatic gastroesophageal reflux disease in newborns as compared with elder children and adults resulted in failure of four clinical trials in infants (esomeprazole, lansoprazole, pantoprazole and omeprazole) [32]. Thus, in newborns, infants and children under 18 months, therapeutic benefit of proton pump inhibitors is not clear and can be limited by subpopulations, for instance, in those diagnosed with erosive esophagitis. In these cases, effectiveness of proton pump inhibitors can be extrapolated from adults along with clinical trials to determine a proper dose and assess safety [28].
Other examples when differences in disease progression could contribute to the inability of pediatric trials to display effectiveness include trials of migraine in adolescents and trials of type 2 diabetes mellitus in adolescents and children. Adolescents have shorter migraine attacks as compared with adults. So, patients can feel spontaneous pain relief during assessment of a typical primary endpoint (i. e., 2 hours after treatment). It hampers demonstration of a statistically significant difference between a drug and placebo. Similar to that, in children and adolescents, 2 type diabetes progresses more rapidly than in adults. This can be associated with a more rapid development of beta cell dysfunction. The real importance of these differences is unclear. Nevertheless, long-term trials have shown that the rate of failure while providing metformin therapy to children with type 2 diabetes mellitus is higher as compared with published data in adults. Moreover, effectiveness in children with type 2 diabetes mellitus failed to be displayed in several trials (glimepiride, rosiglitazone and a combination of fixed doses of glyburide/metformin) [32]. In pediatric patients, high placebo response was a factor leading to a failure in trials of products for therapy of bipolar disorder in children (divalproex) and major depression in children (duloxetine).
The diseases can have a different course in adults and in children. Apart from that, non-correspondent placebo reaction is an additional factor, which can be associated with failures in pediatric trials. Weimer et al (2013) studied placebo effects in children and its causes and concluded that the placebo effect had a negative correlation with age. Thus, in pediatric patients the placebo effect is pronounced to a greater extent than in adults [33].
Drug development for the treatment of rare diseases
Development of drugs to treat rare diseases in children represents a separate and no less important problem. A significant number of people (about 30–40 million European and about 25 million North Americans) suffer from orphan or rare diseases [34]. A single definition of orphan diseases is lacking.
According to Federal Law No. 323-FZ ‘On fundamental healthcare principles in the Russian Federation’, in our country, orphan diseases include diseases with the incidence of at least 10 cases per 100 thousand of people [35]. In the European Union (EU) and Canada, the threshold prevalence includes 5 cases and below per 10 thousand of people [35]. In the U.S., a disease is considered rare when it occurs in less than 200 thousand people [4].
It is important to note that about 80% of orphan diseases are of genetic origin. All the rest is the result of bacterial or viral infections, autoimmune or degenerative disorders. The majority of rare diseases (75%) are manifested within the first 5 years of life [36]. Orphan diseases go through all demographic population groups and all areas of medicine. At the same time, they are often diagnosed during the neonatal period of a child’s development. The majority of rare diseases relate to oncology, oncohematology and neurology. Many rare diseases produce mortality in children. 30% of children do not reach the age of 5 due to the prognosis of fatal diseases and lack of treatment. So, urgent innovations and accelerated drug development are required. While developing drugs to treat rare diseases, three basic principles such as urgency, a limited number of patients and need in complex planning of trials at early stages of development are essential. To improve the dose selection on the accelerated way, clear understanding of accessible information and knowledge gaps is necessary. The type of required therapy (biocorrection or targeted therapy) is of decisive importance. The diseases that need biocorrection with protein and enzyme replacement therapy such as hemophilia or Gaucher disease can be characterized by proper levels of concentrations, physiological pathways and biomarkers.
Nevertheless, as the diseases are rare, complete information about pharmacokinetics and safety, and reliable data about endogenous protein ontogenesis in healthy people can be lacking. As far as targeted treatment methods go, pharmacokinetics and safety data are easy to obtain from trials on healthy volunteers. However, it is more complicated to determine the optimal targeted reach and subsequent extrapolation of data from healthy people to patients. Early dosing data can be obtained from phase I (first-in-man) trials. For instance, the effect of dose on the muscular and adipose mass in programs studying Duchene muscular dystrophy was assessed by way of registration of women in post-menopause during the first-in-man study, as these women, just like patients with Duchene muscular dystrophy have a decreased muscular mass and increased body fat mass. Extrapolation of data from adults to children becomes an essential problem that has to be solved at early stages of clinical trials, especially when the course of the disease in children is different from that in adults, is the principal problem that has to be solved at early stages of a clinical trials.
Data of clinical pharmacology and methods of mathematic modelling can be used to determine the relations between adults and children [37, 38]. Pediatric trials commonly include less subjects as compared with adult trials, whereas a small number of patients with rare diseases just makes the situation more complicated. Innovative plans of trials (adaptive design, Bayesian approach, randomized output method) help adjusting the trial to small groups [4]. Thus, development of drugs to treat rare diseases needs an accelerated process, implementation of innovations, and a reasonable approach to a few patients available. It is stressed that the area needs to develop phase I trial with a large bulk of data, wide use of modelling methods and various sources of information.
Perspectives on pediatric drug development
It should be re-emphasized that it is now the most promising time for development of pediatric drugs. Two international networks of pediatric trials have been developed. Owing to coordinated efforts of the pharmaceutical industry, scientists and regulating authorities, tremendous progress has been made concerning comprehension of age-related changes in drug distribution, especially the drugs associated with oxidation and transportation in the liver [26].
The priority tasks for today include a continued search for non-standard approaches to pediatric drug development and support of an open and reliable dialogue between the interested parties (for instance, regulating authorities, drug developers, academicians, patients and suppliers of medical services) regarding the strategies of pediatric product development and ensuring safe and effective use of drugs in children. Development of pediatric drugs is taken by the world as a global task. Thus, policy and practice of regulating in this area should be agreed upon to the greatest extent. Various activities that promote advanced discussions on pediatric product and trial development, including monthly teleconferences, joint working groups, seminars and expert meetings, are conducted for this purpose [4]. With the introduction of the abovementioned legislative changes, a number of pediatric clinical trials and applications to prolong the patent has increased. As of May 2023, over 1,040 names of drugs were reviewed with addition of data for pediatric use [12]. Moreover, dosing and toxicity data were included for many drugs. Continuous work to support and stimulate pediatric trials resulted in significant success in approval of novel and older off-label drugs in pediatrics.
CONCLUSIONS AND RECOMMENDATIONS
For at least 50 years, it has been stated in patient information leaflets that safety and effectiveness in children were not assessed. It was a legal disclaimer when drugs were used in children. Since the middle of 1990s, regulating authorities of a number of countries have adopted laws, regulatory acts, and compensatory measures for developing companies to increase a number of clinical trials in pediatrics and neonatology. Since the middle of 2000s, a significant growth of these trials has been observed. At the same time, many issues such as ethics, continuity and selection of a dose for trials are still disputable. Newborns belong to a special group, whereas premature newborns are even more vulnerable. Though many global regulating authorities approve the trials in pediatrics, the issue has not been solved yet.
On the one hand, inclusion of children into clinical trials can ensure rapid access to safe and effective drugs for children. On the other hand, participants of pediatric trials can test ineffective or not safe products that will never be approved for or reach the market. Searching for a reasonable balance between these two ethical principles remains relevant even today.